Example Analyses with the 2025 13 TeV Data Beta Release
The general aim of the ATLAS Open Data and tools released is to provide a straightforward interface to replicate the procedures used by high-energy-physics researchers and enable users to experience the analysis of particle physics data in educational environments. Therefore, it is of significant interest to check the correct modelling of several SM process by the 13 TeV ATLAS Open Data MC simulation.
We aim to cover several different analysis categories. This includes searches for the Higgs boson, using different decay channels, and detailed studies of other Standard Model particles such as the W- and Z-bosons and top-antitop quark pairs in final states containing electrons, muons, jets, b-jets or taus. When we are confident that we understand the well-known SM particles the ATLAS Open data can be used to design searches for particles beyond the Standard Model.
The notebooks currently available with the ATLAS Open Data 2025 beta release can be found below. New analysis examples will be added as they are developed and validated, but the examples provided below should be enough to get you started on designing your own favorite analysis!
Example of Physics Analysis
If you prefer to develop your analysis using python inside a Jupyter Notebooks check out the analysis examples provided here.
Top-quark pair production in the single-lepton final state
The top quark is the heaviest elementary particle in the SM, with a mass mt of around 172.5 GeV, which is close to the electroweak symmetry breaking scale. At the LHC, top quarks are primarily produced in quark–antiquark pairs (tt), and due to its large production cross section ( around 830 pb at 13 TeV), the LHC can be viewed as "a top-quark factory". Top quarks have a rich phenomenology which includes high-pT jets, b-jets, leptons and missing transverse momentum. Its understanding is crucial for studying rarer processes, given that tt production is a background to virtually all processes having leptons and multiple jets in their final states.
In order to identify these events, one needs to apply the standard object-selection criteria (defined in "Reconstructed physics objects"), with a stricter lepton pT requirement and tight lepton identification criteria, and an event-selection criteria defined as:
- Single-electron or single-muon trigger satisfied.
- Exactly one lepton (electron or muon) with pT > 30 GeV.
- Missing transverse momentum ETmiss larger than 30 GeV.
- Transverse mass of the W-boson MTW larger than 30 GeV.
- At least four jets with pT > 30 GeV, out of which at least two are b-tagged (with 70% efficiency WP).
At the end, one is able to compare data and MC prediction for the distribution of e.g. the invariant mass of the three-jets combination with the highest vector pT, as seen below:
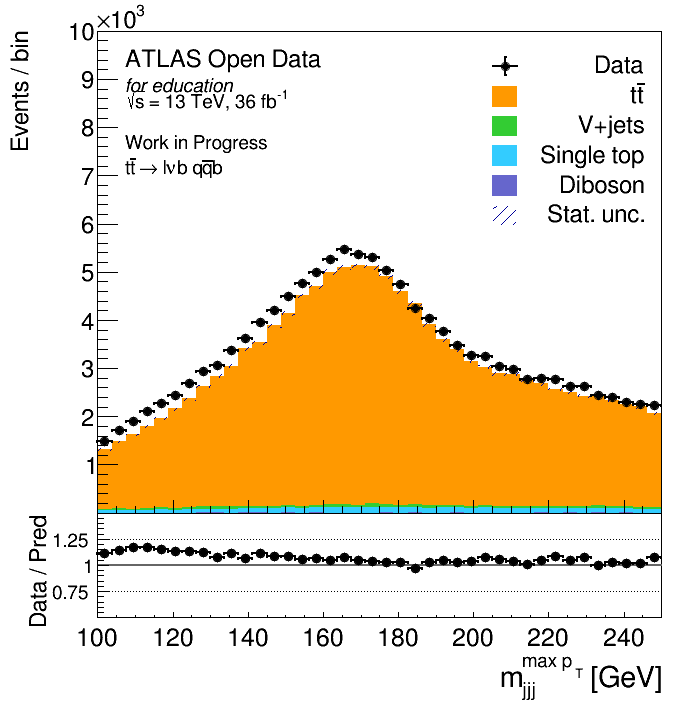
SM Higgs boson production in the H → WW decay channel in the two-lepton final state
As described in the "Brief introduction to the Higgs Boson", the H → WW decay branching ratio for the Higgs boson with a mass of 125 GeV is predicted to be 0.214 in the SM, and corresponds to the second-largest branching fraction after the dominant H → bb decay mode. The predicted Higgs-boson production cross sections via the dominant gluon–gluon fusion (ggF) and vector-boson fusion (VBF) times H → WW branching fraction are 10.4 pb and 0.81 pb for ggF and VBF, respectively. Reducing the numerous backgrounds contributing to this channel and accurately estimating the remainder is a major challenge in this analysis. The dominant background stems from non-resonant WW diboson production, while tt, single-top-quark and W+jets (with the jet misidentified as a lepton) events, as well as non-resonant WZ and ZZ processes contribute to the overall background.
In order to identify these events, one needs to apply the standard object-selection criteria (defined in "Reconstructed physics objects") with a stricter lepton calorimeter and tracking isolation (< 0.1) requirements and tight lepton identification criteria, and an event-selection criteria defined as:
- Single-electron or single-muon trigger satisfied.
- Exactly two isolated, different-flavour opposite-sign leptons (electrons or muons) with pT > 22 and 15 GeV, respectively.
- Missing transverse momentum ETmiss larger than 30 GeV.
- Exactly zero or at most one jet with pT > 30 GeV, and exactly zero b-tagged jets (MV2c10 at 85% WP) with pT > 20 GeV.
- Azimuthal angle between ETmiss and the dilepton system Δφ(ℓℓ,ETmiss) > π/2.
At the end, one is able to compare data and MC prediction for the distribution of e.g. the dilepton transverse mass, as seen below. A small excess in data is observed, and which corresponds to the production of the SM Higgs boson.
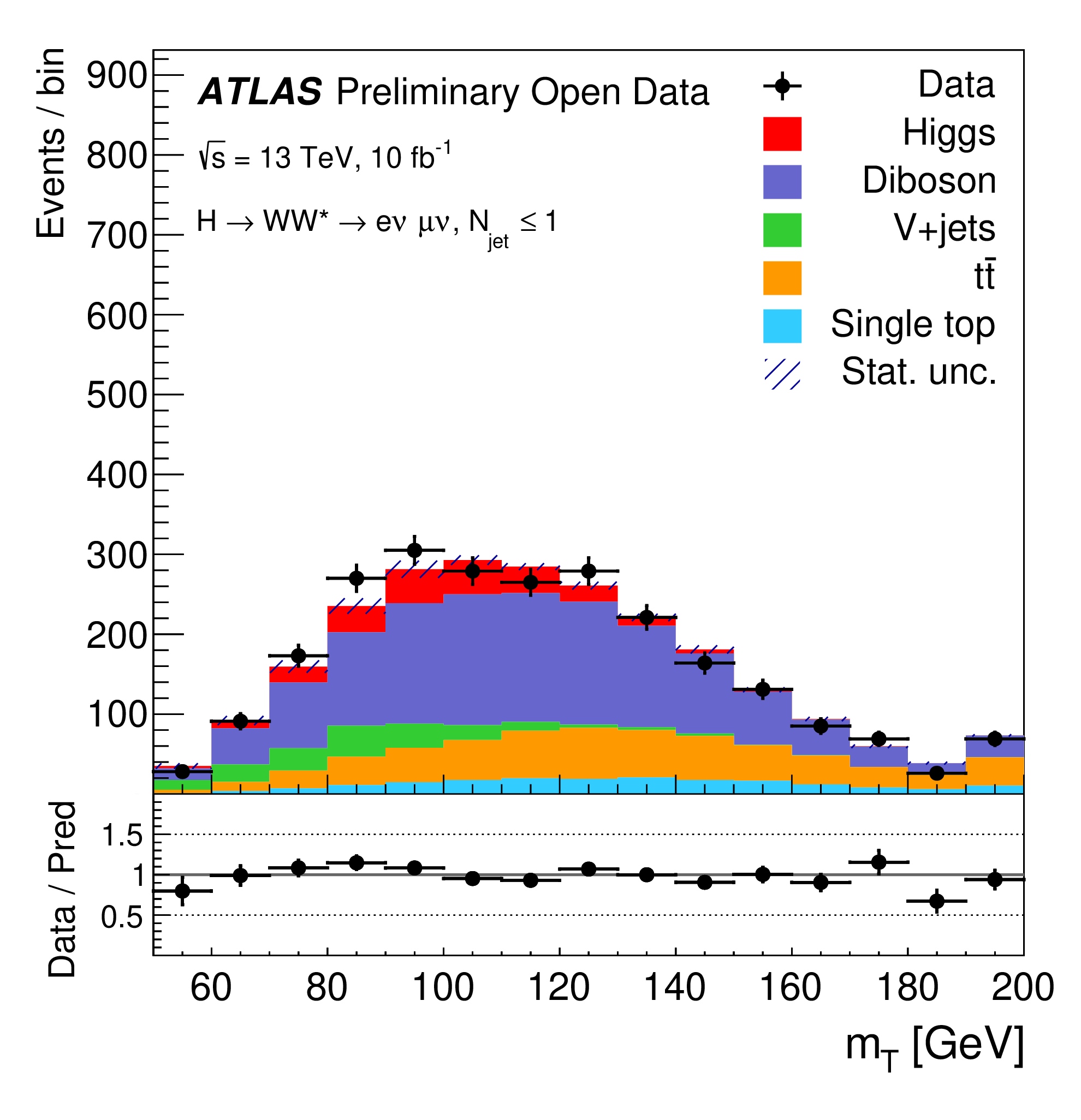
SM Z-boson production in the two-tau-lepton final state
The τ-leptons play an important role in the physics programme of the LHC. They serve not only as a foundation to identify and precisely measure several SM production processes such as W → τν and Z → ττ, which represent the main irreducible backgrounds in measurements of the Higgs boson in H → ττ final states, but also are widely used in searches for new physics beyond the SM.
In the following, a selection criteria is made in order to reconstruct the Z → ττ decays from a hadronically decaying τ-lepton, accompanied by a &tau-lepton that decays leptonically. The leptonic &tau-lepton decays are reconstructed as electrons and muons in the final state. The hadronic &tau-lepton decays produce a highly collimated jet in the detector consisting of an odd number of charged hadrons and possibly additional calorimetric energy deposits from neutral decay products.
In order to identify these events, one needs to apply the standard object-selection criteria (defined in "Reconstructed physics objects") with a stricter lepton pT and lepton calorimeter and tracking isolation (< 0.1) requirements and tight lepton identification criteria, and an event-selection criteria defined as:
- Single-electron or single-muon trigger satisfied.
- Exactly one light lepton (electron or muon) with pT > 25 GeV.
- The two leptons are required to be of opposite charge.
- The transverse mass calculated for the ETmiss and the momentum of the selected light lepton is required to have MTW < 30 GeV.
- The sum of the azimuthal angular separation between the τh candidate and the ETmiss directions, and between the lepton and the ETmiss direction is required to be less than 3.5.
- The visible mass of the τh candidate and lepton, mvis = m(τh,lepton), is required to satisfy 35 < mvis < 75 GeV.
At the end, one is able to compare data and MC prediction for the distribution of e.g. the reconstructed di-tau invariant mass, as seen below.
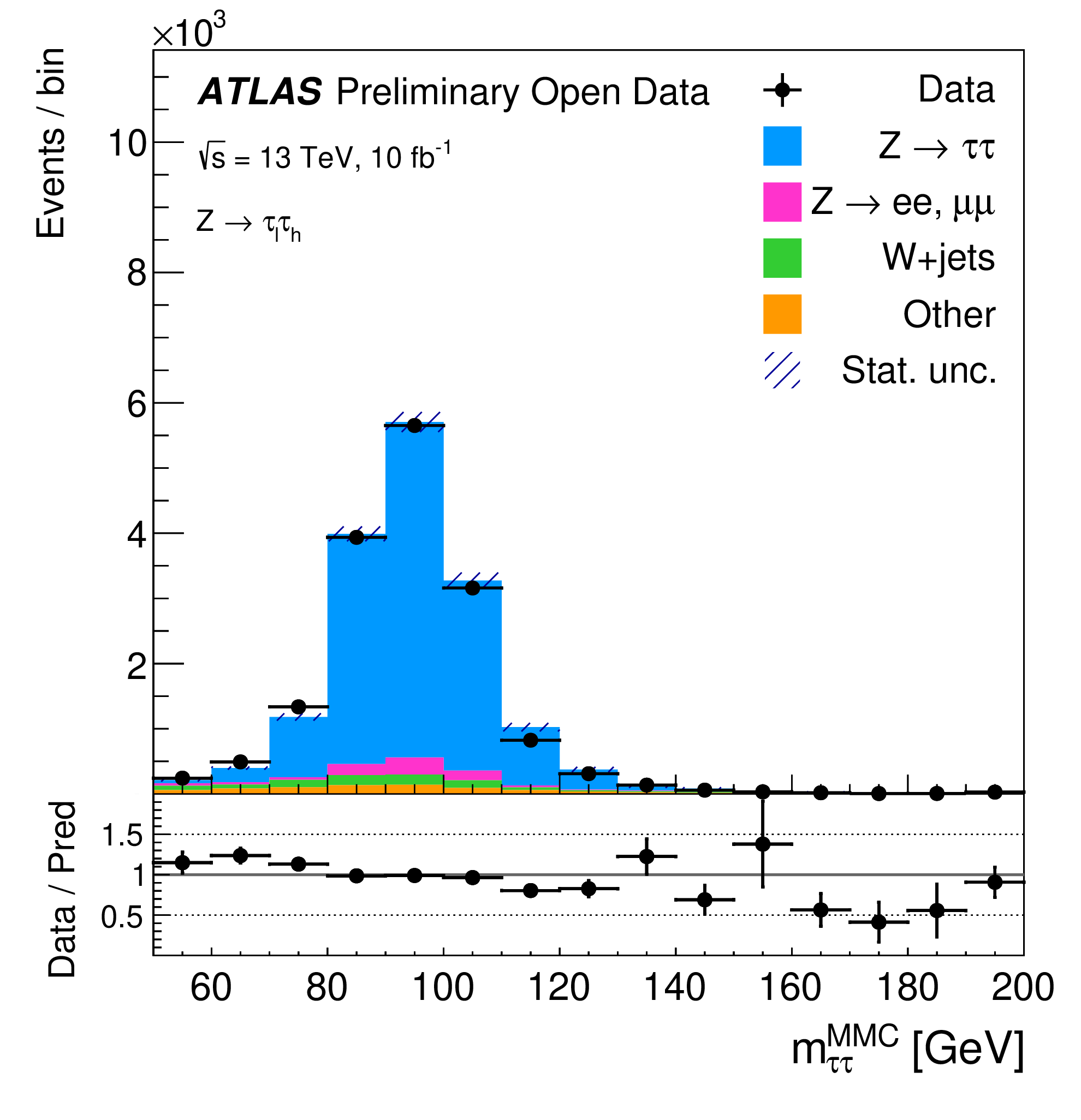